Je suis un physicien théoricien intéressé par la dynamique, la matière condensée et la physique statistique.
Ci-dessous mes sujets de recherche présents et passés.
Interactions et Dynamique à l’Interface Solide-Liquide Nanoscopique
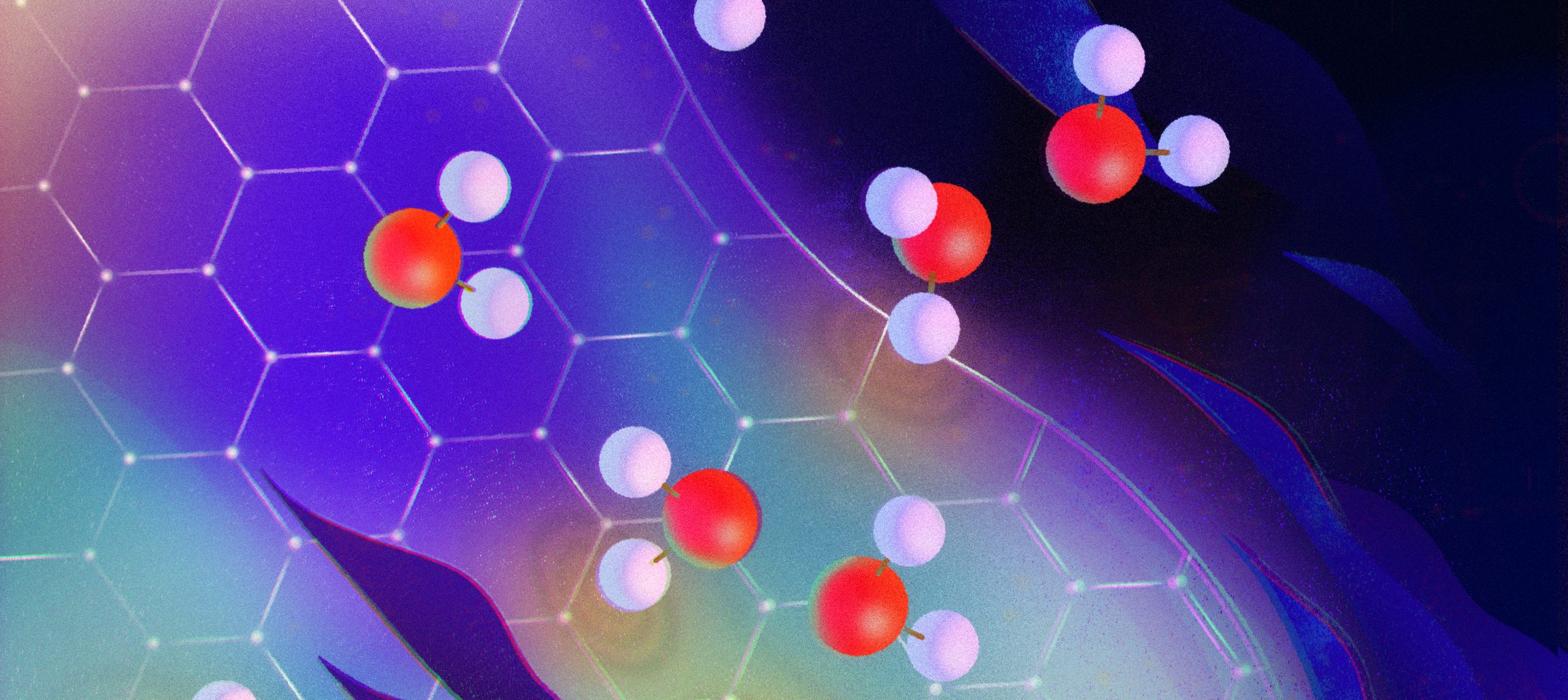
À l’interface solide-liquide, le fluide interagit à la fois avec les phonons et les électrons du solide. Ces interactions, appelées respectivement phonon drag et Coulomb drag, ne peuvent être expliquées correctement qu’en utilisant un formalisme quantique. Alors que les collisions (générant la le phonon drag) sont à l’origine de la friction “classique”, le couplage entre les modes collectifs du fluide et les excitations électroniques à la surface du solide (générant le Coulomb drag) sont la source d’un nouveau type de friction qui est dominant dans certaines conditions de la nanofluidique. En particulier, cela permet de comprendre la friction surprenante dans les nanotubes de carbone où la friction diminue lorsque le rayon est réduit. En imposant un flot, le solide atteint un état de quasi-équilibre où ses différentes particules quantiques vérifient un théorème de fluctuation-dissipation modifié qui inclut un décalage en fréquences. En particulier, nous prédisons la génération d’un courant électrique qui a été mesuré et étudié expérimentalement. La forme du courant est contrôlable en choisissant la structure interne du solide : il s’agit d’un effet quantique. Un tel courant électrique induit par l’écoulement peut avoir des applications révolutionnaires si le solide est bien conçu : cela ouvre la porte à la détection et au contrôle de la vitesse d’écoulement à l’échelle nanométrique et à la production d’énergie à plus grande échelle. Physiquement, le liquide échange son moment avec les phonons et les électrons par le biais du phonon drag et du Coulomb drag, respectivement : c’est comme un vent qui souffle sur la mer de Fermi. Dans la plupart des situations, la première est dominante tandis que la seconde est négative : cela signifie que le moment va de l’écoulement vers les phonons, puis les électrons et enfin vers l’écoulement. En pratique, ce phénomène réduit la friction totale. Cela ouvre la porte à l’ingénierie quantique de la friction en contrôlant la structure interne du solide. De plus, tout ceci peut être affecté par le confinement, ouvrant la voie à de nouvelles découvertes expérimentales.
Références choisies:
[4] Baptiste Coquinot, Lydéric Bocquet, Nikita Kavokine, Quantum feedback at the solid-liquid interface: flow-induced electronic current and its negative contribution to friction, Physical Review X (2023)
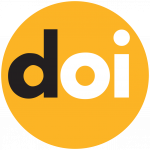
Voir le résumé (en anglais)
An electronic current driven through a conductor can induce a current in another conductor through the famous Coulomb drag effect. Similar phenomena have been reported at the interface between a moving fluid and a conductor, but their interpretation has remained elusive. Here, we develop a quantum-mechanical theory of the intertwined fluid and electronic flows, taking advantage of the non-equilibrium Keldysh framework. We predict that a globally neutral liquid can generate an electronic current in the solid wall along which it flows. This hydrodynamic Coulomb drag originates from both the Coulomb interactions between the liquid’s charge fluctuations and the solid’s charge carriers, and the liquid-electron interaction mediated by the solid’s phonons. We derive explicitly the Coulomb drag current in terms of the solid’s electronic and phononic properties, as well as the liquid’s dielectric response, a result which quantitatively agrees with recent experiments at the liquid-graphene interface. Furthermore, we show that the current generation counteracts momentum transfer from the liquid to the solid, leading to a reduction of the hydrodynamic friction coefficient through a quantum feedback mechanism. Our results provide a roadmap for controlling nanoscale liquid flows at the quantum level, and suggest strategies for designing materials with low hydrodynamic friction.
[3] Mathieu Lizée, Alice Marcotte, Baptiste Coquinot, Nikita Kavokine, Karen Sobnath, Clément Barraud, Ankit Bhardwaj, Boya Radha, Antoine Niguès, Lydéric Bocquet, Alessandro Siria, Strong electronic winds blowing under liquid flows on carbon surfaces, Physical Review X (2023)

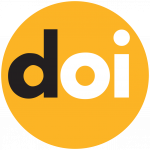
Voir le résumé (en anglais)
The interface between a liquid and a solid is the location of plethora of intrincate mechanisms at the nanoscale, at the root of their specific emerging properties in natural processes or technological applications. However, while the structural properties and chemistry of interfaces have been intensively explored, the effect of the solid-state electronic transport at the fluid interface has been broadly overlooked up to now. It has been reported that water flowing against carbon-based nanomaterials, such as carbon nanotubes or graphene sheets , does induce electronic currents, but the mechanism at stake remains controversial. Here, we unveil the molecular mechanisms underlying the hydro-electronic couplings by investigating the electronic conversion under flow at the nanoscale. We use a tuning fork-Atomic Force Microscope (AFM) to deposit and displace a micrometric droplet of both ionic and non- ionic liquids on a multilayer graphene sample, while recording the electrical current across the carbon flake. We report measurements of an oscillation-induced current which is several orders of magnitude larger than previously reported for water on carbon , and further boosted by the presence of surface wrinkles on the carbon layer. Our results point to a peculiar momentum transfer mechanism between fluid molecules and charge carriers in the carbon walls mediated by phonon excitations in the solid. Our findings pave the way for active control of fluid transfer at the nanoscale by harnessing the complex interplay between collective excitations in the solid and the molecules in the fluid.
Théorie Géométrique de la Mécanique et de la Thermodynamique
Le formalisme usuel pour étudier la dynamique est la mécanique Hamiltonienne qui est basé sur une forme symplectique (ou crochet de Poisson) et une fonction dynamique : l’Hamiltonien. Un tel formalisme est puissant pour étudier la dynamique des objets ponctuels et des fluides dans de nombreuses situations physiques et est bien adapté pour les méthodes numériques. Cependant, la dynamique Hamiltonienne n’inclut pas de dissipation et ne peut donc étudier que les systèmes à l’équilibre thermodynamique. Pourtant, certains modèles dissipatifs peuvent être avec une structure Hamiltonienne en utilisant des formulations exotiques de la théorie symplectique. C’est notamment le cas de la géométrie b-symplectique qui autorise des singularités dans l’espace des phases. Ces situations sont fascinantes d’un point de vu numérique et mathématique et s’appliquent aux systèmes dissipatifs élémentaires. Une autre approche est le formalism métripléctique (ou GENERIC), qui a été développé pour répondre aux limitations des méthodes Hamiltoniennes usuelles. Dans ce formalisme, la forme symplectique est complétée par une métrique pseudo-Riemannienne et l’énergie libre est utilisée comme fonction dynamique. Sous des hypothèses raisonnables, les deux principes de la thermodynamique découlent de la structure géométrique. Un tel formalisme est bien adapté à l’étude des systèmes proches de l’équilibre où la thermodynamique est décrite par la théorie de la réponse linéaire d’Onsager. Ceci s’applique en particulier à la majorité des modèles de dynamique des fluides comme les équations de Naver-Stokes. Dans ces modèles, la métrique (ou le crochet dissipatif) contient la physique microscopique et constitue la réalisation géométrique du tenseur de transport d’Onsager. Elle peut également être dérivée comme une propriété émergente de la théorie des grandes déviations et de la théorie cinétique.
Références choisies:
[2] Baptiste Coquinot, Pau Mir, Eva Miranda, Singular cotangent models and complexity in fluids with dissipation, Physica D (2023)
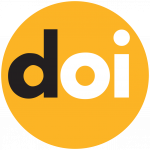
Voir le résumé (en anglais)
In this article we analyze several mathematical models with singularities where the classical cotangent model is replaced by a b-cotangent model. We provide physical interpretations of the singular symplectic geometry underlying in b-cotangent bundles featuring two models: the canonical (or non-twisted) model and the twisted one. The first one models systems on manifolds with boundary and the twisted model represents Hamiltonian systems where the singularity of the system is in the fiber of the bundle. The twisted cotangent model includes (for linear potentials) the case of fluids with dissipation. We relate the complexity of the fluids in terms of the Reynolds number and the (non)-existence of cotangent lift dynamics. We also discuss more general physical interpretations of the twisted and non-twisted b-symplectic models. These models offer a Hamilton- ian formulation for systems which are dissipative, extending the horizons of Hamiltonian dynamics and opening a new approach to study non-conservative systems.
[1] Baptiste Coquinot, Philip J. Morrison, A General Metriplectic Framework With Application To Dissipative Extended Magnetohydrodynamics, Journal of Plasma Physics (2020)

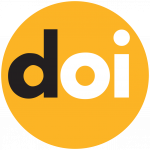
Voir le résumé (en anglais)
General equations for conservative yet dissipative (entropy producing) extended magnetohydrodynamics are derived from two-fluid theory. Keeping all terms generates unusual cross-effects, such as thermophoresis and a current viscosity that mixes with the usual velocity viscosity. While the Poisson bracket of the ideal version of this model has already been discovered, we determine its metriplectic counterpart that describes the dissipation. This is done using a new and general thermodynamic point of view to derive dissipative brackets, a means of derivation that is natural for understanding and creating dissipative dynamics without appealing to underlying kinetic theory orderings. Finally, the formalism is used to study dissipation in the Lagrangian variable picture where, in the context of extended magnetohydrodynamics, non-local dissipative brackets naturally emerge.